A-Level AQA Nuclear Physics Notes - #4
- Sriram A
- Jul 13, 2020
- 7 min read
From the nightmarish detonations of atomic bombs, capable of decimating entire cities, to the astounding energy harvested in nuclear power plants, which could be used to power whole countries, nuclear fission has played a huge part in the development of technology during the past 50 years.
In this post we will be discussing the follow points from the AQA A Level Physics Specification:
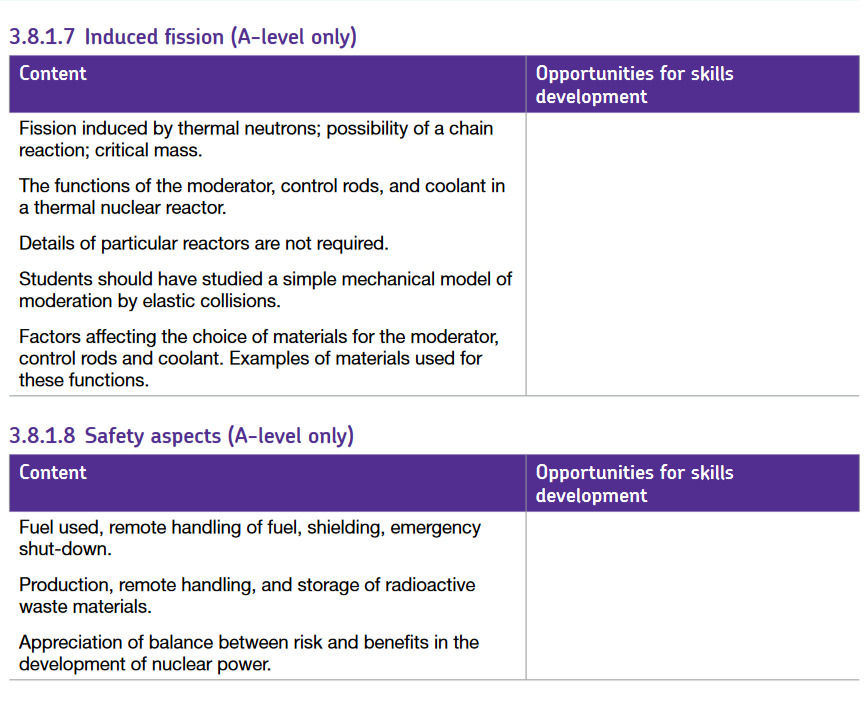
Fission Reactors
Induced nuclear fission occurs when a slow-moving neutron is fired at and absorbed by a stable nucleus e.g. Uranium-235. When the Uranium-235 nucleus absorbs the neutron, it becomes a Uranium-236 nucleus. This Uranium-236 is violently unstable and will decay almost immediately, which is why it is often not shown in nuclear decay equations. This isotope can then decay into smaller nuclei. One of the many decay reactions Uranium-235 can undergo is shown below:

Credits to wikipedia.org
As shown on the far right of the equation, 3 neutrons are produced. These are fast-moving neutrons, which have the potential to cause more fission reactions, hence the term ‘chain reaction.’ During the splitting of the nucleus into the Barium and Krypton elements, an enormous amount of energy is released due to the repulsion of the two positive nuclei. The energy released is the difference in binding energy between the product and initial nuclei. Ultimately, it is this energy that we are trying to harvest in a nuclear reactor.
Although this may sound relatively simple, constructing a device able to exploit these reactions and convert the tremendous amounts of energy produced into useful forms is a complex task.
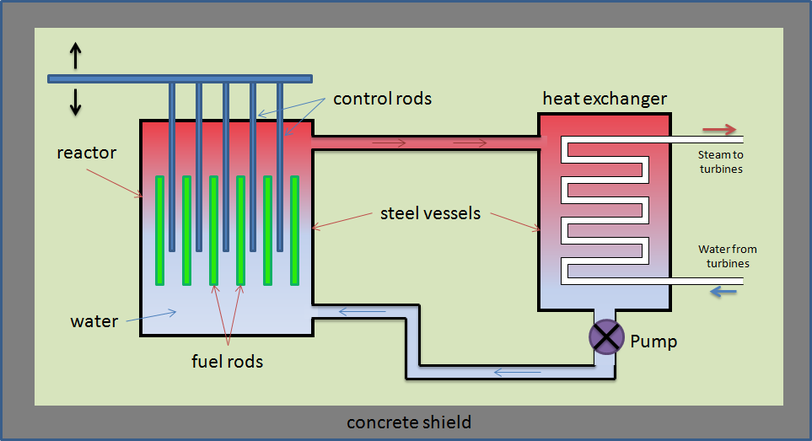
Credits to physbot.co.uk
Shown above is a basic diagram of a thermal nuclear fission reactor. The fission reactions occur in a heavily shielded containment vessel known as the reactor core.
The fuel rods contain the isotopes to be bombarded with neutrons. The fission reactions that occur are known as chain reactions because fast-moving neutrons are produced in each fission reaction, which can cause more fission reactions. For this chain reaction to sustain itself, the mass of fissile material must be greater than a minimum mass, known as the critical mass. This is because some neutrons produced escape from the fissile material without causing fission.
However, there are more neutrons produced per reaction than is required to start a fission reaction. If the fuel was left to react with no means of control, the rate of reaction would grow exponentially, eventually resulting in a catastrophic reactor meltdown due to extremely high temperatures.
To prevent this, control rods absorb some of the neutrons, preventing them from reacting with more fuel. The number of neutrons absorbed is controlled by varying the depth of the control rods in the fuel rods. This is adjusted automatically so that exactly one fission neutron produced by each fission event goes on to cause another fission. This allows for a constant rate of fission, as more neutrons are absorbed. Lowering the rods further decreases the rate of fission, while raising the rods increases the rate of fission, as fewer neutrons are absorbed.
Control rods must be made with non-fissionable materials. This is so that they can absorb excess neutrons without decaying themselves. Boron and cadmium are commonly used for this purpose.
A neutron produced by a fission reaction has too much kinetic energy to be absorbed by other fissile nuclei. This neutron must be slowed down, so it is in thermal equilibrium with its surroundings, to increase the probability of fission. This is achieved by using a moderator.
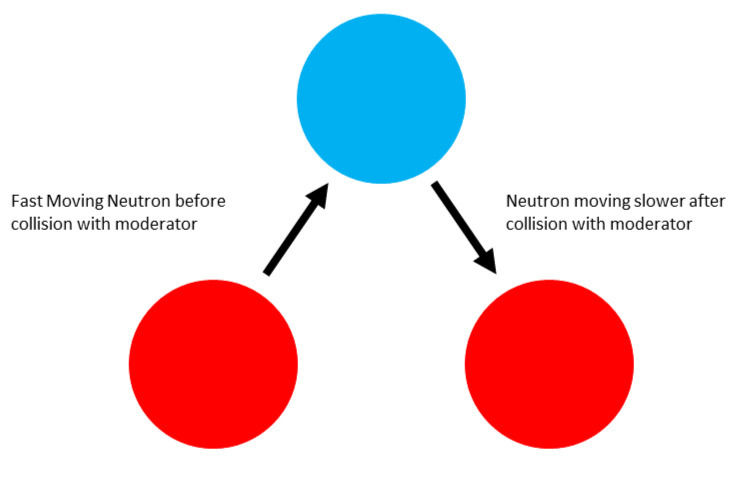
Diagram of collision between neutron and moderator molecule
The moderator is a liquid that surrounds the fuel rods and control rods inside the core. The fast-moving neutrons produced by the fission reactions slow down by colliding with the molecules of the moderator, losing some momentum with each collision. The neutrons are slowed down so that they are in thermal equilibrium with the moderator, hence the term ‘thermal neutron.’
Moderators must be made from light nuclei which are not fissile and will not absorb neutrons. Neutrons must also lose a large energy amount of energy during their collisions with the moderator. Graphite and water are common materials used for moderators.
The energy produced by the fission reactions is entirely thermal. To harvest this energy, a coolant is used. In reactors where the moderator is water, the moderator also acts as the coolant. The coolant captures the heat produced and transfers it to a heat exchanger through a pressuriser. The heat exchanger contains a pipe filled with water which is heated up by the coolant. This water then evaporates and is transferred as steam to turbines, which generate electricity. The coolant, after exchanging heat with the water from the turbines then cools down, and enters a pump, which forces the water back into the reactor core to repeat the process.
Coolants must be able to transfer heat easily and must be fluids that can fill the core. The coolant must also be chemically stable at high temperatures and must not be corrosive. Water is a very common coolant due to its high heat capacity and availability.
Safety Aspects
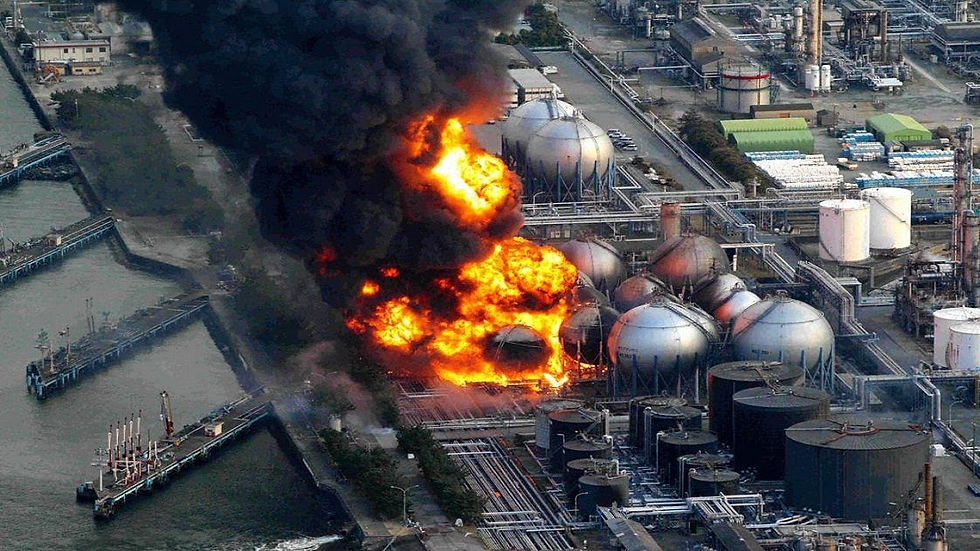
Aerial Image of the Fukushima Daiichi Nuclear Power Plant shortly after three reactor core meltdowns and hydrogen explosions
While it may seem like this energy is available at little expense, there are many consequences to the use of nuclear fission reactors. These can be mitigated through safety features implemented in the reactor.
Fission reactors produce deadly amounts of radiation, which could cause serious damage if it were to reach the environment. To prevent this, the reactor core is designed as a thick steel vessel that can endure the extremely high temperatures and pressures in the core. It also absorbs beta radiation and some of the gamma radiation and neutrons. The steel vessel is shielded by very thick concrete, which will absorb any neutrons and gamma radiation which escapes from the reactor vessel so that almost no radiation is leaked outside the reactor.
In the event of a malfunction, the control rods are lowered completely into the reactor core to prevent any further fission reactions from occurring. This prevents a disastrous nuclear meltdown from a runaway chain reaction, such as those that have occurred in the past at the Chernobyl Nuclear Power Plant and the Fukushima Daiichi Nuclear Power Plant.
Workers who maintain these power plants handle the fuel rods with remote devices to avoid contact with radioactive materials. Before use, fuel rods contain Uranium-238 enriched with Uranium-235. These fuel rods only emit alpha radiation. This is absorbed by the fuel cans containing the rods. After use, the fuel rods emit large amounts of beta and gamma radiation due to the neutron-rich nuclei that are formed. Spent fuel rods also contain Plutonium-239 which is highly carcinogenic as a very active alpha radiation emitter.
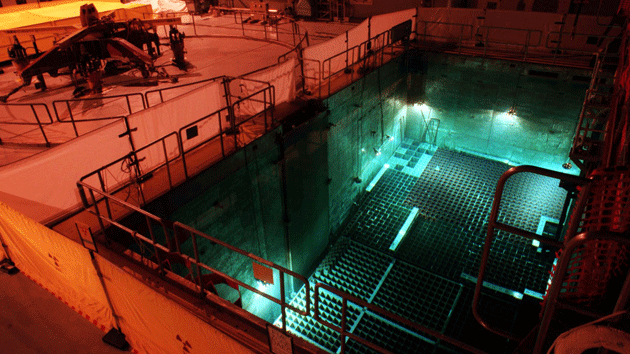
The spent-fuel pool at Duane Arnold Energy Center in Palo, Iowa
Various protocols have been made for the handling of radioactive waste.
High-level radioactive waste is produced from spent fuel rods. These must be removed remotely using heavy shielding and stored underwater in cooling reservoirs temporarily as they continue to release heat from ongoing radioactive decay. They are then taken to reprocessing plants to remove unused uranium and plutonium for possible further use. The remaining material is radioactive waste. This material is normally stored in sealed containers deep underground. It must be stored securely for very long periods to prevent contamination of food and water supplies.
Intermediate-level waste may be generated from nuclear fuel cladding, chemical sludge, or materials contaminated during the decommissioning of a nuclear power plant. These materials do not require cooling and are instead stored in drums encased in concrete, which are sealed into the walls of special buildings made from reinforced concrete.
Low-level waste is generated from hospitals and industry and has very small amounts of radioactivity. While some active materials may require shielding, low-level waste is generally sealed in metal drums and buried in shallow trenches.
Nuclear fission reactors have many advantages to traditional fossil fuel sources. They have very low greenhouse gas emissions, almost comparable to renewable energy sources like solar and wind power. Nuclear fuel also has a very high energy density compared to fossil fuels, with Uranium-235 having an energy density of 79.4 mega Joules per kilogram. Furthermore, nuclear fission is not intermittent, unlike wind and solar farms; power plants can run for a year without interruption.
However, despite the many safety features implemented, when a nuclear reactor does go wrong, it can have devastating repercussions on the surrounding areas. Nuclear power plants also produce a large amount of nuclear waste which is still a major issue, as people do not want spent nuclear fuel to be stored in their localities. Nuclear power plants can also be very expensive to build, with initial starting costs reaching up to 7 billion pounds.
If we are to consider nuclear energy as a possible main source of energy to combat climate change and power more developing countries, then all these factors must be considered.
To check your understanding of nuclear reactors and their safety aspects, please attempt the following questions. Full explanations are given below.
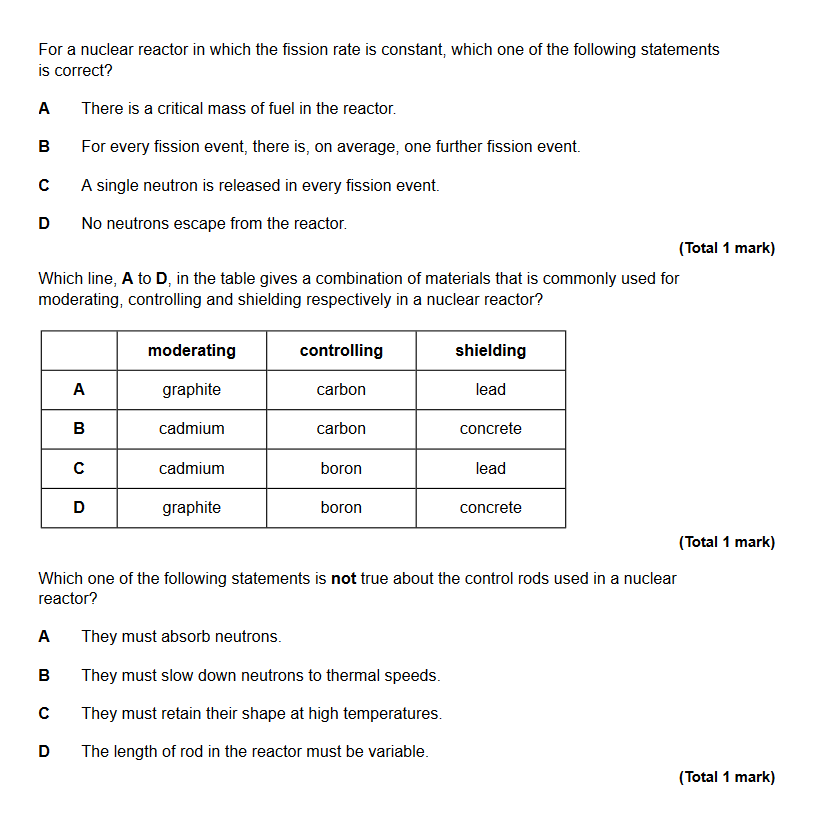
Question 1- B
The fission rate is constant so there must be, on average, one further fission event caused by each fission event.
We do not know if A is true as we are not given whether the reaction is sustaining itself.
C is not true as we know that in a fission event multiple neutrons are released.
D is not true as it is not possible for there to be absolutely no neutrons escaping from the reactor.
Question 2 - D
Graphite slows neutrons down readily and is light. Boron absorbs neutrons without undergoing fission. Concrete is a dense material which absorbs radiation very well.
While the materials given for the moderator and shielding are right, A is wrong as carbon cannot be used for control rods, as it can absorb neutrons and become fissile.
B is wrong as cadmium is used for control rods, not moderators. And again, carbon cannot be used for control rods.
C is wrong for the same reasons B is wrong, despite boron and lead being reasonable control rod and shielding materials respectively.
Question 3 - B
Control rods do not slow down neutrons to thermal speeds; moderators do this.
A is true as control rods absorb neutrons to control the rate of fission.
C is true as control rods are subjected to extremely high temperatures and are directly adjacent to the fuel rods.
D is true. The length of the control rods in the core is variable to control the rate of reaction and stop the reactions completely in the event of an emergency shut-down.
Thank you for reading this series about nuclear physics. If you have further questions about any part of this series feel free to contact us, either by email or on the Subject Help forum. If you are not a member of OnlyPhysics, please consider joining us - it's completely free and comes with loads of benefits!
Comments