Introduction:
Since the Wright brothers first took to the air in 1903, there has been plenty of discourse as to how they - and everyone after them - managed to get a heavier-than-air object off the ground, or more specifically what mechanism allow their wings to generate lift.
There are two main theorems regarding airfoils creating lift, both of which I shall describe, along with their drawbacks. I will then go on to describe what I believe the best explanation as to how wings allow these iron beasts to traverse the sky.
The Theories
First, the Bernoulli Theorem, or Bernoulli Effect:
Here is a standard cambered airfoil - cambered essentially means non-symmetrical (photo credit Alamy).

The curved upper surface of the wings leads to air molecules travelling faster over them compared to the flat(ish) bottom surface; it's very difficult to explain - most sources brush over this as a fact of experience - but imagine a ball on a spring hung from the ceiling, and an airfoil like the one above passing just under it, pushing it upwards as it slides over the top. The spring will push the molecule down, which relative to the movement of the airfoil means it is technically moving faster.
Not only is this quite a bad analogy, it is probably completely wrong. An aeronautical engineer would most likely feel violence toward me for this - but ask me in a year or so, and I'll replace it with a less offending one, promise.
With regards to lift, the faster moving molecules over the top create a lower pressure relative to below - this is because there are less molecules at any given time, and due to P = nRT/V, P ∝ n, n being the number of molecules (again, this is probably grossly oversimplified - forgive me). Lower pressure above creates a differential in force (shown by P = FA), with the molecules on the bottom pushing up on the wing - that's lift!
This theorem is all well and good until we get to cambered airfoils working perfectly fine upside down! This only works under one certain condition, which leads to me to the second issue below.
That problem is symmetrical airfoils, like those used on the stunt plane below, photographed by Tom Claud.

Here, there is no difference in velocity, so no pressure differential leading to lift. So if the Bernoulli Theorem does not explain lift, how is this thing in the air?
The answer lies in Newton's Third Law - whenever two objects interact, they exert equal and opposite forces on each other.
There are several ways of explaining this phenomenon, and my favourite is through momentum. Let's use a massively simplified diagram, courtesy of yours truly, as you may perceive from the spider that crawled across the page. We have a line at 45 degrees, which is travelling horizontally through still air - it's easiest to show this using the line as a frame of reference, meaning we see the air travelling towards a still line. If you haven't already got there, this is meant to represent a symmetrical airfoil at an acute angle of attack (usually it would be less than 20 degrees to the horizontal or we'd be stalling, but that's a problem for another day).
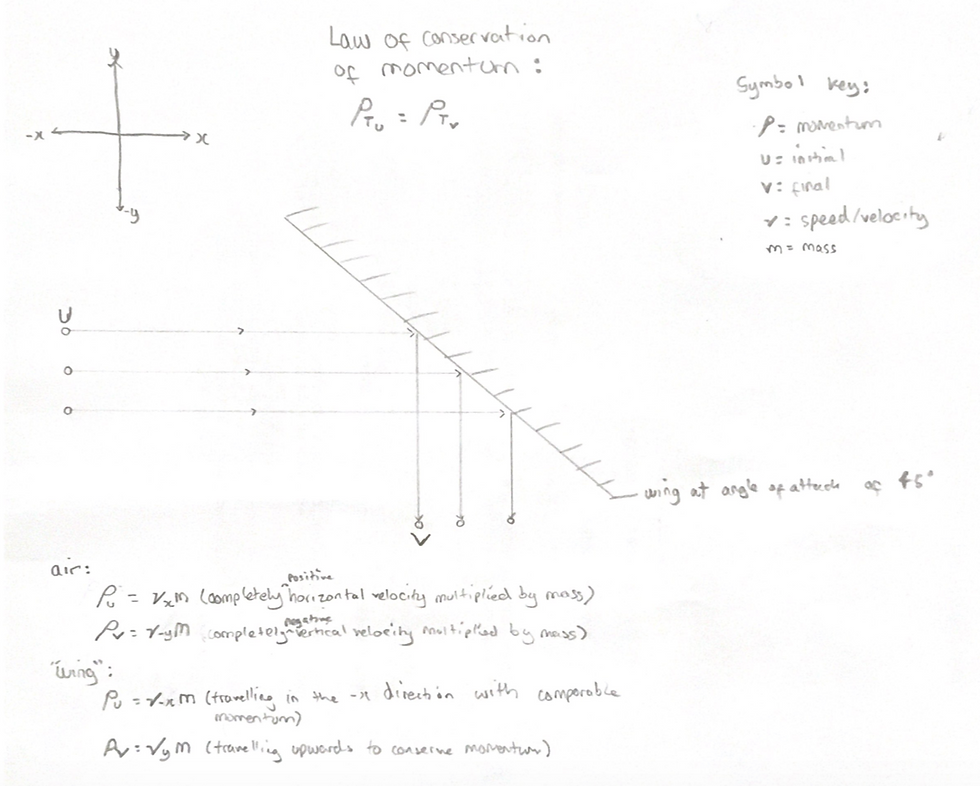
If it wasn't clear enough from the diagram, the air moving in the x-axis is redirected to the y-axis. This introduces a new direction for momentum to be conserved in, which is vertically. The air is moving downwards, so the wing must move upwards. Therefore, the conservation of momentum means an angled wing must create lift.
This is not how things work in reality of course - wings will be at no more than a 20 degree angle of attack under normal operation, so air molecules will not be redirected straight down, but more deflected slightly downwards, which means there is both a horizontal and vertical component of momentum - in the instant of this example, the plane is actually rising straight up, which would remove all horizontal velocity, causing a stall. Additionally, wind is an issue here - planes flying into the wind will have a lower groundspeed (speed relative to the ground, funnily enough) as their true airspeed (which is what stall speed is compared to) is higher, due to more air molecules coming into contact with the wing at a given time - essentially, it's almost like the plane is flying faster. This is why landing into the wind is beneficial for short runways, where the plane can fly very slowly but still stay airborne due to the air creating artificial wind.
A far simpler way of looking at this is that a flat-ish object pointed at an acute angle and moving horizontally will naturally be pushed up. If this isn't immediately obvious, next time you're in the car hold your hand out of the window facing the direction you are going in and point it to the sky a little - you'll feel it pushed up (please do this responsibly - I accept no liability if an oncoming truck gives you a hi-five).
With regards to taking off, which was an issue I had to think about for a moment, symmetrical airfoil planes would either already be at an angle due to higher nose gear than rear gear, or have wings already angled, although this would slightly defeat the purpose of symmetrical airfoils, which is primarily versatility.
Problems with this explanation are limited for this discussion to the fact that it does not explain pressure differences recorded in flight that are explained by the Bernoulli effect.
This theory also explains why cambered planes can fly upside down; they just have to fly at a higher angle of attack. Moreover, it would explain why cruising passenger planes will fly at a small angle of attack - they gain the benefit of this as well as the Bernoulli effect, which makes the flight more fuel efficient. And there's even more - the momentum theory explains one cause of the benefits of a manoeuvre carried out by fighter pilots and space shuttles known as aerobraking, where the angle of attack is raised significantly, so the plane/spacecraft is doing the aeronautical equivalent of a car skidding along on its rear bumper, which causes it to brake heavily. In regard to momentum, it reflects air molecules back with a component in the x-axis, meaning the plane must decrease velocity to maintain momentum (a side effect will naturally be an increase in lift). This is only carried out at high speeds or a very real risk is run of stalling (still another day for that conversation).
Anyway, I digress. These are the two main explanations for how a wing generates lift, along with interesting side notes and one or two issues that they are subject to.
The Answer
So, let us return to the question - which theory allows an aeroplane to fly? Well, the answer is: both. Both phenomena are equally valid empirically, if not theoretically (one is certainly more explained than the other). Newton's Third Law in terms of lift will be present in almost every flight you see (I say almost because I don't want to make any bold claims about something I cannot know for sure), and Bernoulli's Theorem in all cambered airfoil flights, like those made by passenger planes like the A320 below.
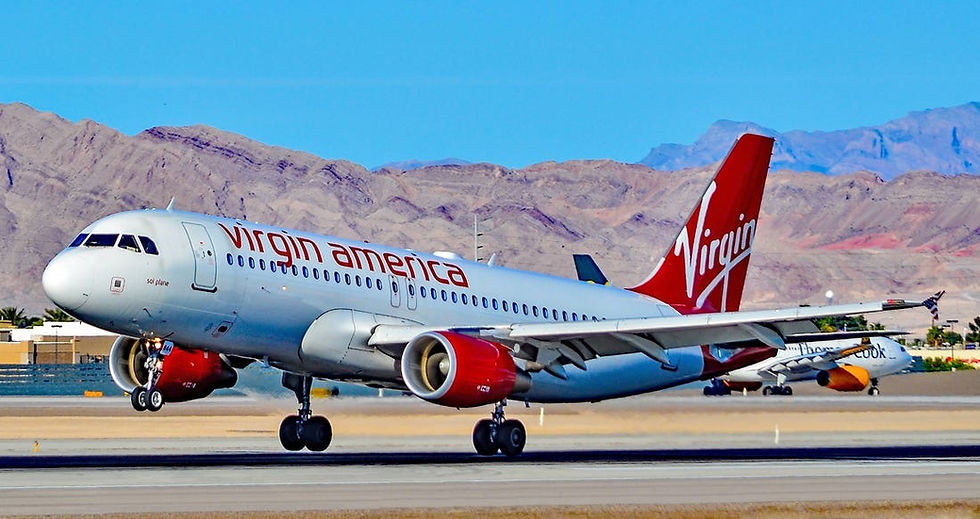
Taking the example of a passenger plane, here is how the two interact:
Takeoff: Bernoulli Effect allows nose to rise, creating angle of attack, allowing N3L (thanks for the abbreviation of Newton's Third Law, Mr Beadling!) to come into play (disregarding any original nose raise from landing gear design)
Climb: Both theories are equally valid, with N3L potentially being more significant due to questions surrounding the Bernoulli effect taking place at 100% efficiency with non-whole components of forces when an airfoil is at an angle - these are largely left alone, but I may do some research for a future article if it looks promising.
Cruise: As previously touched on, the plane will be at a small angle - roughly 2.5 degrees for a Boeing 737 like the one below. Therefore, both effects would be equally important here for efficiency - the more work can be left to the laws of physics, the less the jets have to do!
Descent: Here, for passenger comfort and safety (and to avoid overspeeding which can be catastrophic), pilots have a specific process for this that I will avoid like the Black Plague. Both theories are still in play.
Landing: With flaps extended, there is a nice large wing for the N3L theory to affect, which means it is quite significant - however, the Bernoulli Effect is as always still important.
Conclusion
So how do aerofoils work? In a nutshell, the answer lies in a combination of pressure differences as a result of the shape and in the conservation laws of physics, which introduce a vertical force when there is a non-zero angle of attack.
Now, there are numerous other explanations for lift, albeit not all this readily accessible, so my disclaimer is that this is by no means a definitive article, and that there are plenty of people who will offer equally valid reasons for why planes fly.
In any case, I think that will draw us to a close - thank you for reading this post. If you enjoyed this article, please consider joining OnlyPhysics - it's completely free and we post plenty of other blogs on so many interesting areas of academia, as well as offering free subject support. Thanks!
Kommentarer